Resistomatic - an Arduino-based autoranging ohm-meter
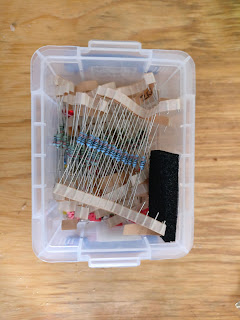
Sorting resistors is a pain If you've been having fun with electronics for a while you've probably got a box with bits in . I've been playing with electronics for six decades and I have lots of boxes, many of them containing random collections of resistors. Resistors are cheap, but I hate waste, so from time to time I decide to empty some of the boxes and put the resistors away in their proper homes. It ought to be easy, but it's a pain. I was never good at reading colour codes, and the problem has got worse with age. What I want is a simple, quick autoranging ohm-meter that I can use to measure resistor values. So I'm making resistomatic . Resistomatic requirements Most of the resistors I use are 1% or 5% tolerance metal film resistors in the range 220 Ohms to 100K Ohms. Almost all the values are in the E12 range. (E12 values are multiples of 1.0, 1.2, 1.5, 1.8, 2.2, 2.7, 3.3, 3.9, 4.7, 5.6, 6.8, 8.2). I'll be happy if resistomatic can achi